

Copied from Figure 1 of the publication ( 20) ( b) the backbone of our 3D model derived from this secondary structure, colored to match the coloring of the three pseudoknots. Our 3D model of it, depicted in Figure 6b, proves that it is, and thus provides a basis for detailed, atomic-level studies of structure-function issues.ĬrPV IRES modeling: ( a) secondary structure and an associated cryo-EM density map (colored pink) of the IRES bound to the 40S ribosomal subunit of HeLa cells. For instance, if one considers the proposed base-pairing scheme shown in Figure 6a of the Cricket Paralysis Virus internal ribosome entry site, it is not obvious that it is 3D-realizable. Thus, we wished to know if the information is 3D-realizable that is, whether there is a stereo-chemically correct 3D model of a conformation consistent with this information. Initial motivation for the program was primarily that of testing base-pairing information of large RNAs, however they it may have been derived.

Extrapolating from this partial 3D information to a complete, specific, stereo-chemically correct 3D conformation constitutes the general problem addressed by the program.
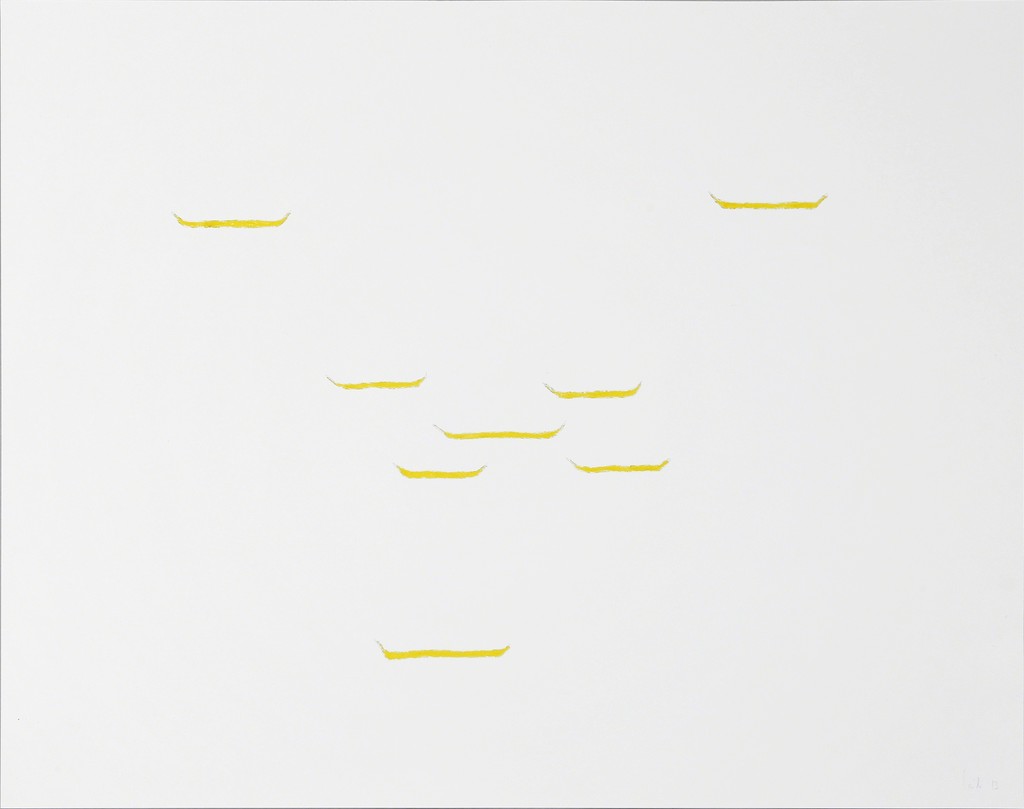
The base-pairing structure of an RNA molecule thus gives a general idea of its 3D conformation in that it determines how some of the coordinates are related to one another.

Base-pairing not occurring in stems relate to the tertiary structure of the molecule. These double-stranded helices (hereafter referred to as stems), constitute the secondary structure of the molecule. Successive base-pairs serve to identify 3D structure motifs in the form of double-stranded helices. But its Watson-Crick base-pairing secondary structure can, by a combination of experimental, phylogenetic, and energy-based computational techniques, be determined with reasonable accuracy. Illustrative applications in the areas of ribozymes, viral kissing loops, viral internal ribosome entry sites, and nanobiology are presented.Ĭurrently, there is no algorithm for computing the entire 3-dimensional structure of a relatively large (greater than approximately 45 nts) RNA molecule from its primary structure (nucleotide sequence). Previously determined coordinates for any part of the molecule are readily incorporated, and any part of the modeled structure can be output as a PDB or XYZ file. Subsequent refinement of the first-order approximation, of modifications, and for the imposing of tertiary constraints is assisted with standard energy refinement techniques. Compaction, whereby base stacking within stems is optimally extended into connecting single strands, is also available as a means of strategically making the structures more compact and revealing folding motifs. With emphasis on fast exploration of alternative 3D conformations, one may interactively add or delete base-pairs, adjacent stems can be coaxially stacked or unstacked, single strands can be shaped to accommodate special constraints, and arbitrary subsets can be defined and manipulated as rigid bodies. Applicable to structures of arbitrary branching complexity and pseudoknot content, it features efficient interactive graphical editing for the removal of any overlaps introduced by the initial generating procedure and for making conformational changes favorable to targeted features and subsequent refinement. Using primary and secondary structure information of an RNA molecule, the program RNA2D3D automatically and rapidly produces a first-order approximation of a 3-dimensional conformation consistent with this information.
